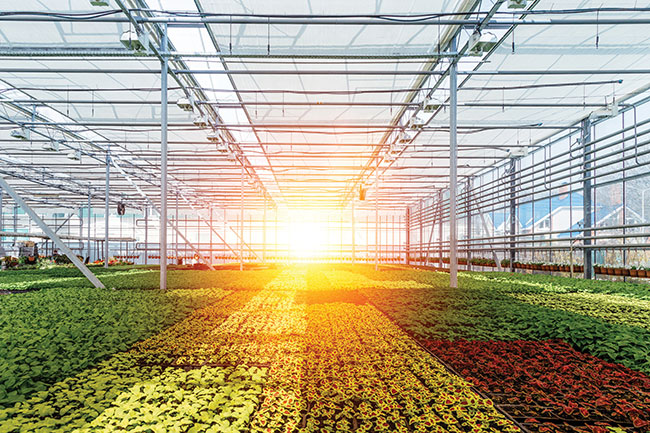
Striving for optimal greenhouse control
After labor costs, energy is typically the second largest expense in greenhouse crop production. Mechanization and automation can help reduce labor requirements as long as the technology is cost-effective. The economics of such technology investments will depend on the number of units that need to be processed and the resulting quality of the end product.
Finding the right solutions can be challenging, especially when unanticipated outside factors (e.g., politics, war, pandemic) directly or indirectly impact greenhouse operations. While the choices between labor and technology are important, this article will focus on strategies growers can use to reduce the energy inputs needed to successfully grow greenhouse crops.
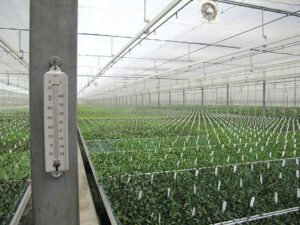
TEMPERATURE CONTROL
Plants perform best when their environment is maintained within an optimal temperature range. This range can vary depending on plant species and variety and involves the temperature of the surrounding air, the temperature of the canopy and the temperature of the root zone. For convenience, many greenhouses are controlled based on the air temperature because it is relatively easy to get an accurate reading.
But the greenhouse air temperature typically varies depending on where the measurement is taken (it is often cooler near ventilation inlets and warmer closer to the roof) and is therefore not always a good representation of what the crop experiences. Therefore, sensor location is an important factor. In most greenhouses, the volume of air inside is much larger than the volume taken up by the crop. Thus, using the air temperature to control the heating and cooling systems may result in additional costs compared to the case where the control decisions are based on information about what the crop is experiencing.
For example, controlling the greenhouse environment based on the crop canopy temperature may result in lower energy costs and higher crop quality. While measuring the canopy temperature is a little trickier than measuring the air temperature, equipment is available from various vendors that can accurately report this information.
Similarly, knowing the root zone temperature can inform about plant status and, if controllable, can be used for optimal control at minimal costs. There is a large variety of equipment and approaches available for controlling the key greenhouse temperatures. The best solutions depend on crop requirements, cost, reliability, grower preferences and maintenance requirements.
HUMIDITY CONTROL
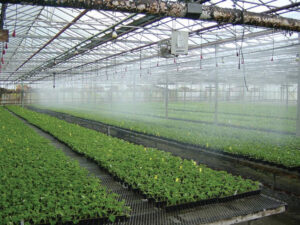
Humidity control of the greenhouse air is closely tied to air temperature control. Warmer air can hold more moisture (water vapor), causing the relative humidity to go down (assuming no additional water is evaporated into the air as it warms). Like temperature, plants perform best when the humidity of the surrounding air is maintained within an optimal range.
When the surrounding air is too dry, plants lose more moisture to the point that they begin to wilt. High humidity levels make it more difficult for plants to transpire (reducing water and nutrient movement from the roots to the shoots and limiting the plant’s ability to cool itself) and can increase the risk of fungal diseases.
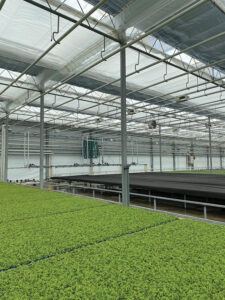
Adding more moisture to the greenhouse air is relatively straightforward — a misting system is effective and relatively low-cost. Removing moisture from the greenhouse environment can be done by replacing humid air with drier air (e.g., through ventilation) or by removing the moisture through a cooling process (colder air can hold less moisture so it will condense out during the cooling process). The latter can be expensive when technology such as mechanical refrigeration is involved.
While traditional humidity control has focused on maintaining specific relative humidity values, from the plant’s perspective it makes more sense to focus on the control of the vapor pressure deficit (VPD). VPD is the difference between the saturated vapor pressure of the air inside the stomatal cavities (i.e., at the canopy temperature) and the partial vapor pressure of the surrounding air (which can be calculated using the temperature and relative humidity of that air). It’s the VPD that determines that plant’s ability to move water vapor to the surrounding air and is therefore a better indicator of plant status than the relative humidity of the surrounding air.
LIGHT CONTROL
Solar radiation (consisting of ultraviolet, visible and infrared radiation) and supplemental lighting (if used) provide the energy source for photosynthesis. We use the instantaneous intensity and the daily light integral (DLI) across the Photosynthetically Active Radiation (PAR) waveband to quantify the impact of light on plant growth and development.
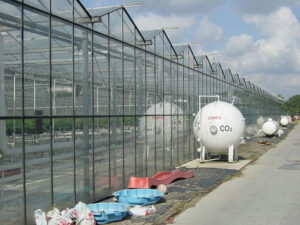
Supplemental lighting requires electricity and can be expensive due to the large number of fixtures needed to deliver an adequate intensity and/or DLI. As a result, accurately controlling the amount of light needed is key to maintaining optimum plant growth and development, while minimizing electricity costs. There are times of the day/year when greenhouse crops receive too much solar radiation, requiring the use of shade curtains. A sophisticated light control system relies on measurements of the light reaching the crop canopy in order to operate the supplemental lighting and shading system.
Depending on the light fixtures installed, the intensity and/or spectral output can be adjusted based on the desired light targets. The latter is only practical when light-emitting diode (LED) fixtures are used. The newest LED fixtures are more energy efficient compared to the traditional high-intensity discharge (metal halide and high-pressure sodium) fixtures but are more expensive to buy. The (waste) heat LED fixtures produce is also a little easier to deal with than the radiant heat produced by high-intensity discharge fixtures. Return on investment calculations are needed to determine whether it makes sense to invest in the latest lighting technology.
CARBON DIOXIDE CONTROL
In addition to light (energy) and water, carbon dioxide is the third input for photosynthesis. Therefore, each of these three inputs can become the limiting factor for photosynthesis. The concentration of carbon dioxide in the air is very small (on average 420 parts per million as of this writing) and plants growing in an enclosed environment such as a greenhouse can quickly deplete the available carbon dioxide.
Because carbon dioxide is colorless and odorless, we need sensors to tell us what the concentration is. Such sensors are especially important when we use supplemental lighting. If the carbon dioxide concentration is insufficient to maintain a high rate of photosynthesis, running the supplemental lighting system will not result in more plant growth because the carbon dioxide concentration is too low. Increasing the carbon dioxide concentration can be accomplished by burning natural or propane gas in specially designed burners, or by vaporizing liquefied carbon dioxide.
Growing crops that have a large amount of biomass during the winter months when ventilation rates are often low usually requires carbon dioxide enrichment so that the rate of photosynthesis remains high (assuming light and water are not the limiting factors). Research has shown that enriching the greenhouse environment with carbon dioxide to a concentration of 1,000-1,200 ppm can be beneficial during times when the ventilation system is inactive. At those concentrations, there is no negative impact on humans working in such an environment, but for safety reasons, an alarm system should be installed that can warn people when the concentration exceeds 5,000 ppm.
INTERACTIONS
It would be nice (from a control point of view) if the environmental parameters impacting plant growth and development were independent, but that’s not the case.
Changes in air temperature impact the humidity and the rate of photosynthesis, as do changes in light intensity and carbon dioxide concentration. Because greenhouses are designed for maximum light transmission and are therefore not well insulated, the indoor environment experiences substantial fluctuations that have to be counteracted by the control system.
The more we impose stringent control set points, the more expensive it will be to achieve them. If, on the other hand, we can relax the control approach (e.g., by aiming for an average temperature over a specific time period as opposed to a fixed temperature, or by moving supplemental lighting to times of the day with lower electricity rates), we may be able to save substantially on the overall operating costs.
I recommend trying novel approaches in a small growing area before deciding on implementing changes for an entire operation. Consulting with crop advisors, researchers, Extension personnel, equipment suppliers and fellow growers can provide useful insights.